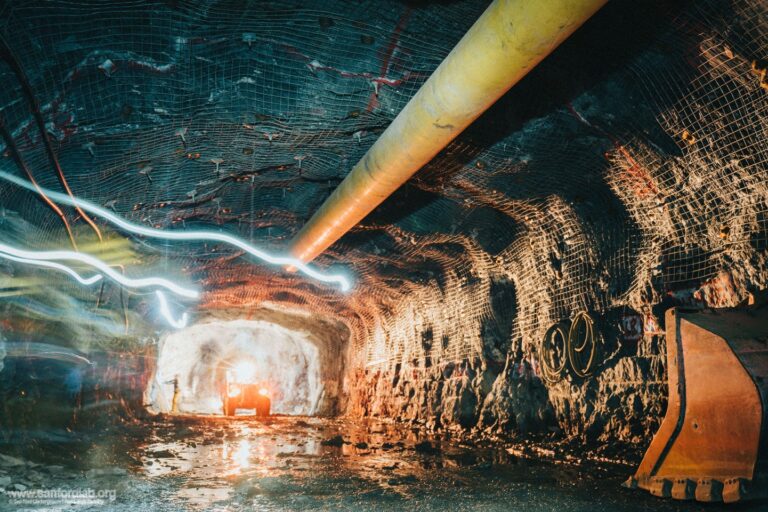
[ad_1]
WASHINGTON, D.C.—U.S. physicists have a bold new plan to “explore the quantum universe,” from the smallest bits of matter to the broadest reaches of the cosmos.
On December 7 the Particle Physics Project Prioritization Panel (P5), a committee of experts that convenes roughly once a decade, debuted its draft report, which charts a course for U.S. particle physics across the next two decades. “What we are really after is: ‘How does the universe work?’” says Hitoshi Murayama, chair of P5 and a theorist at the University of California, Berkeley.
To further this fundamental search, the P5 report made a number of key recommendations, including a crucial cost-saving compromise for the Deep Underground Neutrino Experiment (DUNE), a troubled $3-billion effort in South Dakota that is presently the nation’s largest particle physics project. The 164-page report also offered strong support for building the Cosmic Microwave Background Stage IV (CMB-S4) project—a series of telescopes to study the early universe—and for pursuing R&D for a muon collider, a technically intimidating goal that could pay off with discoveries of new particles and interactions.
The recommendations have drawn a largely positive reception, despite including some cuts. “We are delighted about the report,” says Lia Merminga, director of Fermi National Accelerator Laboratory (Fermilab) in Batavia, Ill. “We enthusiastically believe it provides an ambitious vision and road map.”
For proponents of a muon collider, it was like Christmas come early: Language in the report called for a “muon shot” akin to the “moonshot” of the U.S. Apollo program in the 1960s. “I am thrilled,” says Isobel Ojalvo, an experimental particle physicist at Princeton University. Ojalvo and other early career researchers are excited about a muon collider, she says, “not only because it is a paradigm shift in particle physics but also because it is a hard problem to solve.”
On Monday hundreds of researchers gathered at Fermilab, the locus of U.S. particle physics. They discussed the report and its implications, with hours of questions and comments ranging from technical scientific queries to concerns about the report’s formatting.
After the questions ended, the physicists made their way to a reception where Murayama and Merminga cut into a cake decorated with a clear message: “SHOOT FOR THE MUON.”
Open Questions
Throughout the past century, physicists have used experiments of increasing energy and precision to uncover how the universe works at the smallest scales. Their efforts have led to the Standard Model of particle physics, which describes the most fundamental ingredients of matter (leptons and quarks) and the forces that control them (the electromagnetic, strong and weak forces). At the same time, scientists have also developed a standard model of cosmology to account for the largest scales. This is a recipe for the evolution of the universe, from the beginning of time to today (and beyond): Start with the big bang. Add one part dark matter, two parts dark energy and just a dash of matter. Let simmer for circa 13.8 billion years.
Of course, the extraordinary explanatory success of these models doesn’t mean physicists have run out of open questions about the cosmos. The new P5 report organized several areas of inquiry into “science drivers,” including the study of neutrinos—particles that interact feebly with matter—and of dark matter—the invisible material that gravitationally dominates galaxies and other gigantic cosmic structures. Whereas previous P5 reports focused on small scales, this one harkened back to the big bang to underscore the unity of all things and the profound connections between small and large: “Remarkably, the entire observable universe, now billions of light years across, was once small enough to be quantum in nature. Its quantum history is imprinted on its large-scale structure,” the latest report stated.
Informed by years of community discussions, known as “Snowmass” (after Snowmass, Colo., where the meetings used to be held), the P5 committee deliberated and whittled down the myriad suggestions into 20 recommendations to the Department of Energy and the National Science Foundation (NSF), the two major funders of U.S. particle physics.
Despite its visionary scope, the P5 committee’s most pressing issue was arguably to course correct a project already well underway: DUNE, the overbudget and behind schedule U.S. flagship particle physics experiment. DUNE is a giant detector being built in a South Dakota mine to receive a stream of neutrinos beamed from Fermilab through a 1,300-kilometer-long stretch of continental crust. DUNE’s planners say that studying how the neutrinos change in transit should allow it to pin down the elusive properties of these notoriously slippery particles, especially the question of whether they behave differently from their antimatter counterparts. According to DOE officials, after years of delays the excavation for DUNE’s main cavern is now 90 percent complete, and the experiment should receive neutrinos in 2031. That’s too close for comfort for some critics, who note that DUNE’s competitor, a Japanese experiment called Hyper-Kamiokande, will start operations in 2027—early enough to potentially beat the U.S. project to the punch on key neutrino measurements.
To minimize further costly delays, the P5 report recommends streamlining DUNE by curtailing the project’s upgrade plans—an intervention that could save nearly $1 billion, the committee says. “Do we really need a 2.4-megawatt beam? Do we really need a fourth detector? Putting everything together, it didn’t look like it,” Murayama says. Although some physicists at the Fermilab town hall voiced concerns about P5’s cuts making DUNE less competitive with Hyper-Kamiokande, Murayama is sanguine. “Just relax, and do the best job we can do,” he says.
Cosmic Choices
In line with its lofty emphasis on studies of the large-scale universe, the P5 report gave its highest priority to CMB-S4, an $800 million project to study the cosmic microwave background—the first light of the universe visible after the big bang—using a dozen ground-based radio telescopes. Jim Strait, a physicist at Fermilab and director of CMB-S4, calls the endorsement “a vote of confidence” in the project’s importance and plan.
Rigorous planning is crucial for CMB-S4 because the experiment’s telescopes will operate in some of the world’s harshest, most remote regions—in Chile’s desolate, high-altitude Atacama Desert and at the Amundsen-Scott South Pole Station in the heart of Antarctica. Both locations rank high on the list of Earth’s darkest, clearest skies, but the South Pole site in particular poses resource and transportation challenges. If Strait and his team can manage the logistics—which include securing an unwavering source of funding—CMB-S4 should start peering into the early universe in the opening years of the 2030s.
The P5 report also endorsed upgrades to an existing Antarctic experiment, the IceCube Neutrino Observatory. Using thousands of sensors buried in the ice, the detector spots flashes of light from impinging high-energy neutrinos from across the cosmos. P5 recommended a plan to expand IceCube’s volume 10-fold, which would also boost the number of neutrinos the detector sees by a factor of 10.
Over the past decade, dark matter experiments looking for Weakly Interacting Massive Particles (WIMPs) have come up empty-handed. The negative results have pushed WIMPs off their pedestal and broadened the search for the nature of dark matter. The P5 report recognized this shift and recommended a new WIMP search only in the case of budgetary largesse. Instead the report pushed heavily for a number of smaller projects looking for non-WIMP dark matter. “Smaller-scale experiments will survey the wider set of dark matter theories,” it stated.
Clashing Colliders
Negative results have also affected plans for future particle colliders. After decades of theoretical prediction were borne out by the 2012 discovery of the Higgs boson, which confirmed how other particles get their mass, CERN’s Large Hadron Collider (LHC) has failed to find any other new fundamental particles.
As part of its continuing study of the Higgs, this particle accelerator will be upgraded to become the High-Luminosity LHC (HL-LHC), which will start up in 2029. Although the HL-LHC will operate at the same energy as the LHC, the upgraded version of the particle accelerator is projected to collect 10 times more data than has been gathered so far. The P5 report supported continued funding for the HL-LHC but without the explicit addition of auxiliary detectors that would capture otherwise-unseen unusual particle decays. One proposed suite of auxiliary experiments, the Forward Physics Facility (FPF), would have relied on such detectors to search for neutrinos, dark matter, and more sourced from LHC collisions.
While FPF is not dead—CERN may still choose to build it, absent U.S. support—Jonathan Feng, a physicist at the University of California Irvine, who proposed the project, laments the P5’s decision. “Without [FPF], you see no neutrinos,” he says. “With the FPF, we’ll see thousands each day. It’s a way to let us see LHC collisions with completely new eyes.”
In the previous P5 report, issued in 2014, an electron-positron collider ranked second after DUNE on U.S. physicists’ wish list. (Positrons are the antimatter counterparts of electrons.) Such a machine would yield clean, lower-energy collisions that would be ideal for creating and studying Higgs bosons—making it a so-called Higgs factory. (The protons the LHC uses are made from a jumble of quarks and gluons, and their messier collisions obscure studies of the Higgs.) The 2023 P5 report still recommended an internationally-based Higgs factory—but under limited budgets, it suggested delaying funding in favor of more urgent priorities.
This diminished appetite for a Higgs factory is partly because of uneasy prospects. The International Linear Collider (ILC) topped the 2014 collider wish list and is “shovel-ready”—it could be built right away in Japan. Years of indecision among Japanese politicians have led many physicists to conclude it is dead, however. CERN’s bid for a Higgs factory, the Future Circular Collider (FCC), would require a 90-km tunnel dug near Geneva, wouldn’t be operational until nearly 2050 and would not necessarily be a huge improvement over the HL-LHC—all of which has led to anxiety about the proposal, especially among U.S. physicists.
Meanwhile China’s recent disclosure of progress toward a homegrown Higgs factory may have dealt another blow to prospects for the ILC and the FCC alike: at a physics conference in Germany last month, Chinese particle physicists confirmed rumors that the nation’s proposed Higgs factory, the Circular Electron Positron Collider (CEPC), could be approved and start construction as early as 2027. The P5 report did not specify whether the U.S. would provide funds for CEPC, given the tense relationship between the country and China.
High Hopes for Higher Energies
Fallout from the lack of new particles at the LHC has also pushed physicists toward higher energies. Specifically, they’d like a machine that smashes particles to probe the 10 tera-electron-volts (TeV) energy realm, an order of magnitude increase from that of the current LHC that many researchers hope is the threshold beyond which new phenomena will appear. (For perspective, 1 TeV is the approximate kinetic energy carried by a flying mosquito.)
Enter the muon. The heavier cousins of the electron, muons could offer high-energy collisions via surprisingly compact hardware. Though particle physicists have toyed with muons as collider ammunition for decades, the idea has recently taken root—especially among U.S. physicists—for two main reasons. First, a new wave of design studies showed that building a muon collider is likely feasible despite the fact that muons live a scant 2.2 microseconds, making them much harder to harness than longer-lived protons and electrons. Second, a muon collider offers a chance to reach 10 TeV in the U.S. on a shorter timescale than that proposed by most potential international competitors. A 10-TeV muon collider would be only 16 km in circumference—conveniently able to fit snugly inside the boundaries of Fermilab.
The last time the U.S. attempted to build a new high-energy collider, things did not go well. The Superconducting Super Collider (SSC) would have been an 87-km ring on the Texas prairie that would reach energies three times higher than that of the LHC. In 1993 it was killed by Congress partway through construction, partly because of mismanagement and budget overruns. Since then the SSC’s fate has haunted particle physicists and led to caution around big projects.
The P5 report did not explicitly mention the SSC, but by calling for a path to a muon collider, it rebuked the specter of the dead megaproject. “By taking up this challenge, the US blazes a trail toward a new future,” the report argued. “This is our Muon Shot.”
[ad_2]